Origin of batteries
Electrochemical fuel cells are composed of three components: a negatively charged cathode, a positively charged anode and an electrolyte that creates a chemical reaction which moves electrons in a circuit. Eventually the chemistry in the fuel cell neutralizes, and the circuit is lost. We need a process that enables an electrical circuit to reverse the chemistry in the fuel cell to recharge it. This happens in the 12-volt lead-acid batteries found in most automobiles, as an alternative provides a reversing electrical current. The problem with lead-acid batteries is their size, weight, and acid material. To this end, researchers have developed a smaller rechargeable cell, of which the lithium-ion battery is currently the most efficient. These are the batteries we find our mobile phones and increasingly in electric vehicles; hybrid automobiles today typically use rechargeable nickel metal hydride fuel cells.)
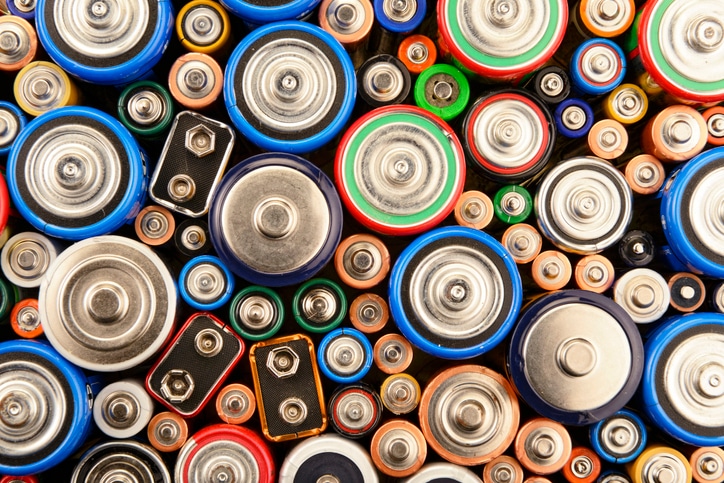
Various batteries
Lithium ion battery components
When you discharge a lithium-ion fuel cell, positively charged lithium ions move from a negative electrode (anode), commonly graphite (C6), to a positive electrode (cathode) that forms a lithium compound with cobalt oxide (LiCoO2). During charging, the opposite occurs with the graphite attracting lithium ions to form LiC6. Both electrodes enable lithium ions to move in and out of their structures through an electrolyte and a separator diaphragm.
While lithium-ion batteries have enabled better charging/recharging properties of batteries, considerable research is happening by battery vendors and academics to improve the density of energy the cells carry, the operating temperatures, and the safety. They are also working to increase the lifespan and decrease charging times while eliminating expensive cobalt materials.
Lithium ion battery analysis techniques
Each component of the fuel cell is a construct of engineered materials. In the case of smaller batteries found in microelectronics, these features are quite small. Fortunately, materials scientists have at their disposal a number of analytical techniques to characterize the lithium-ion (LiO) fuel cells at the bulk, microscopic, and multilayer scale. Each technique provides specific insight into the composition and construction of LiO fuel cells, forming a holistic approach to creating more efficient cells, and their improving the quality control.
Even the most pristine material for LiO fuel cells may contain impurities that rob the battery of its performance, including particles of various sizes, shapes, and distributions made up of chromium, iron, copper or nickel which can interrupt the electrical flow of the fuel cell, even causing internal shorts.
Scanning electron microscopy
For quality control, our Phenom ParticleX Desktop Scanning Electron Microscope (SEM) with integrated Energy-Dispersive Spectroscopy (EDS) automates the ability to locate, study distribution and characterize the morphology of contaminant particles in samples. With EDS, the ParticleX SEM enables quality control specialists to identify the elemental and chemical composition of the particle in order to perform a root cause analysis of the contamination such as locating the source of the contaminant in the production workflow. ParticleX automation provides the ability to prepare sample batches to run overnight, using a step-and-repeat process to microscopically examine industry-standard filters to locate, measure and classify any contaminant particles for remedial actions.
Raman microscopy
Raman microscopy, which combines vibrational spectroscopy with light microscopy, examines the chemistry and structure of LiO fuel cells down to the micron scale. Raman reveals information about the molecular composition and crystalline structures of materials. A Raman microscope can look into the layers of a material without destroying the sample, saving it for further analysis by other techniques. Imaging software allows the researcher to create a chemical map across an area of the sample, noting structural and chemical changes, and identifying contaminants. Raman is also a valuable technique in identifying carbonaceous materials such as graphite and graphene, which can play roles in fuel cells.
Our DXR3xi Raman Microscope takes advantage of special sampling chambers that allow a researcher to transfer LiO components from a glovebox to the microscope without oxidizing the sample and corrupting its chemistry. We also use a specialized electrochemical test cell that enables the researcher to observe chemical changes during charging and discharging cycles.
Micro computed tomography
As the chemistry and structure of the fuel changes during charging and recharging cycles, the ability to look at the cell in three dimensions proves invaluable. Micro computed tomography (MicroCT) uses X-ray to non-destructively cross-section materials to recreate a 3D model of the material. Our HeliScan MicroCT System uses advanced helical scanning and iterative reconstruction technology to produce a 3D image at the fuel cell level. MicroCT can be an initial step in a multi-scale, multimodal workflow that progresses through higher-resolution imaging with a focused ion beam/scanning electron microscope to atomic-scale analysis in a transmission electron microscope.
Focused ion beam scanning electron microscopy (FIB-SEM)
Looking at the individual components in the cell, DualBeam (FIB-SEM) instruments provide highly localized characterizations of materials by combining the precise sample modification of a focused ion beam (FIB) with the high-resolution imaging of SEM. This technique is ideal for studying cathode and anode samples to provide a representation volume distribution, microstructural quantification, and characteristics such particle cracking, phase boundaries and binder distribution around electrochemical materials at the component and particle scale.
Software for lithium ion battery analysis
Our Avizo Software provides advanced image processing and segmentation techniques ideally suited for this multiscale 3D imaging. At the macro level, Avizo Software can be used to assess leakage, porosity, or delamination. It can also examine aging processes by monitoring the quality of the foil, cathode, and anode. At the microscopic level, Avizo Software allows for the estimation of the tortuosity and permeability of the porous electrode and separator. Effective transport parameters and the cell’s performance can be further analyzed with electrochemical performance simulations as well as quantification of the triple phase boundary (TPB), phase distribution and connectivity.
X-ray photoelectron spectroscopy
X-ray photoelectron spectroscopy (XPS) measures the signal from photoelectrons energized in the XPS system, which are weaker in energy than other technique signals and provide information regarding surfaces and layers of just a few nanometers in thickness. This enables researchers to study how species form at interfaces in the material composition.
Thermo Scientific XPS systems are useful for comparing used and unused cathode and anodes and can characterize metals, oxygen and carbon, binder materials and any electrolyte material on the surface of an electrode. In a recent XPS experiment, comparison of the major elements (Mn, Co, Ni) showed little difference between uncharged and charged cathodes. However, examination of the used cathode revealed a considerable reduction of lithium in the used sample. This experiment quantified the electrochemical migration of lithium from the cathode to the anode after charging.
Like electron microscopes, XPS systems can be equipped with an ion source that profiles into the bulk of the sample. Comparing a manufactured anode material with an aged anode, we see that with aging the surface interface between the anode elements has evolved into the bulk of the material, including a build-up of halogen-containing compounds, which could indicate the build-up of SEI or the breakdown of the binder material.
When looking at layers and surfaces of only a few layers of atoms, using an ion beam to mill into the sample is likely to change the structure or chemistry of the sample. While standard ion milling tools use Argon ions to etch a material, instruments such as our K-Alpha XPS system include a monatomic ion source. This ion source uses clusters of low energy Argon ions (1eV – 100eV) that cause minimal surface penetration, mitigating damage to the surface chemistry. The MAGCIS monatomic ion source includes large Argon clusters that are ideal for etching organic materials found in a fuel cell’s layers, and small clusters to interrogate metal oxides and alkali metals.
Gas cluster ion techniques for battery analysis
Gas cluster ion techniques enable the investigation of new candidate materials for fuel cells such as lithium phosphorus oxynitride (LiPON) layered on a Si substrate which would be chemically altered using traditional ion etching. XPS using the cluster ion beam are also useful for understanding interfacial reactions relating to the solid electrolyte interphase (SEI) layer in LiO cells. The role of the SEI layer involves the prevention of further electrolyte decomposition to maintain cycling ability. Studies have shown that adding a sub-nanometer thickness coating has been found to inhibit the rate of SEI layer growth on electrode surfaces.
To help lithium ion battery manufacturers and researchers understand the analytical capabilities offered by Thermo Fisher, we recently conducted a webinar presentation that covers many of the techniques highlighted in this article.
Resources
Webinar: Lithium Ion Battery Analysis
Video: Advancing Lithium-Ion Battery Technology with 3D Imaging
Website: Battery Research Enhanced with Electron Microscopy and Spectroscopy
Website: Particle Analysis with Electron Microscopy
Want to speak to someone? Need a quote? Want a demo? |
Opt in for email communications on this and other topics. |
Leave a Reply