Transmission electron microscopy and its increasing use in semiconductor applications
Scientists have been working for many decades to develop higher magnification microscopes to drive forward scientific progress. In 1924, Louis de Broglie published his revolutionary theory of electron waves. Shortly thereafter, with the still-novel understanding that the wavelength associated with electrons was much smaller than the wavelengths in the visible light spectrum, physicist Ernst Ruska invented the first electron microscope—launching the history of TEM as we know it.
Since then, electron microscopy instrumentation has proliferated in many industries, especially the semiconductor industry which relies on scanning electron microscopes (SEMs) for “in fab” defect analysis and metrology. However, as fabricated devices have grown in complexity, requiring higher resolution data on 3D structures, the use of transmission electron microscope (TEM) systems has grown significantly.
In this blog post we’ll go over the fundamentals of TEM technology and its semiconductor applications. It will be the first in a series of new Thermo Fisher Scientific educational TEM content for semiconductor professionals.
Watch the TEM fundamentals webinar on-demand >>
First, a look at the history of TEM instruments and some of their key components
In Fig 1 you can see the recent decades of TEM development and the shift from a focus on imaging resolution reduction, to more productivity and the acquisition of higher quality, more repeatable data, particularly for semiconductor applications.
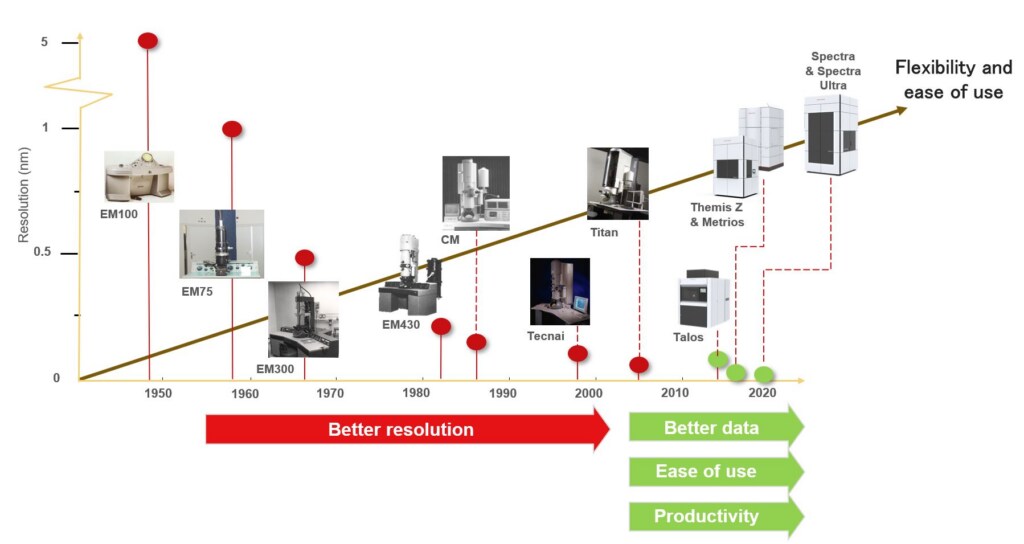
Fig 1: A summary view of the last 70 years of TEM development
Transmission electron microscope components
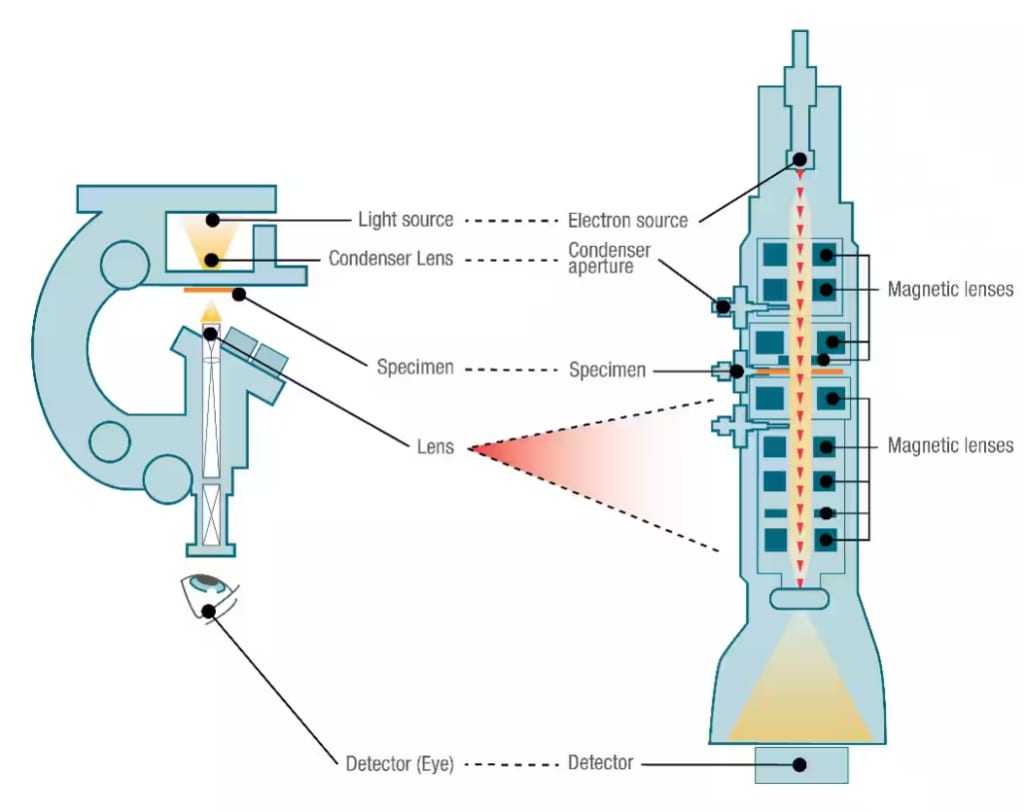
Fig 2 : An inverted image of a traditional benchtop microscope (left) highlights the similarities to a TEM (right).
How does a TEM work? Much like a benchtop optical microscope that projects light through a thin sample which can then be viewed by the human eye—with magnification by lenses, of course—a TEM directs a beam of electrons through an ultrathin sample. After it passes through the sample, the transmitted beam then interacts with a variety of filters, detectors and cameras below. A TEM can resolve details as small as individual atoms, giving access to invaluable structural and elemental information from features which could be buried deep within a sample.
TEM has evolved significantly from its early days as a complex tool used by researchers and scientists. Though today’s instruments are highly automated and considerably easier to use, the language used on this topic and the sheer number of acronyms involved can be quite daunting. For example, you will see references to TEM, STEM, (S)TEM and S/TEM in articles such as this. What is the difference and what does each term mean?
Starting at the beginning, TEM is the fastest and often simplest mode of operation for a (S)TEM instrument. The application involves shooting a focused beam at the sample and then collecting the transmitted electrons in a single “snapshot” on a high-speed, high-resolution camera underneath the sample, as shown in Fig 3 below.
How does a TEM work? Much like a benchtop optical microscope that projects light through a thin sample which can then be viewed by the human eye—with magnification by lenses, of course—a TEM directs a beam of electrons through an ultrathin sample. After it passes through the sample, the transmitted beam then interacts with a variety of filters, detectors and cameras below. A TEM can resolve details as small as individual atoms, giving access to invaluable structural and elemental information from features which could be buried deep within a sample.
TEM has evolved significantly from its early days as a complex tool used by researchers and scientists. Though today’s instruments are highly automated and considerably easier to use, the language used on this topic and the sheer number of acronyms involved can be quite daunting. For example, you will see references to TEM, STEM, (S)TEM and S/TEM in articles such as this. What is the difference and what does each term mean?
Starting at the beginning, TEM is the fastest and often simplest mode of operation for a (S)TEM instrument. The application involves shooting a focused beam at the sample and then collecting the transmitted electrons in a single “snapshot” on a high-speed, high-resolution camera underneath the sample, as shown in Fig 3 below.
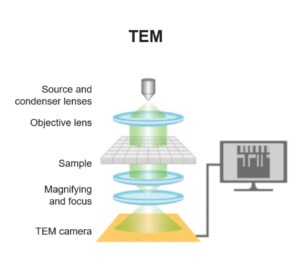
Fig 3: Transmission electron microscopy – “TEM mode”
Alternatively, a (S)TEM can be operated in STEM mode shown (as below in Fig 4), where a precisely focused beam is scanned across the sample by the use of electromagnetic “scan coils.” The transmitted electrons are then captured on a variety of STEM detectors below the sample and reconstructed into a data set. The number and location of the STEM detectors enables extremely flexible, high contrast imaging data to be acquired, even on complex semiconductor structures.
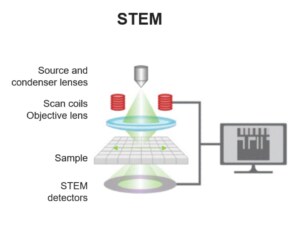
Fig 4: Transmission electron microscopy – “STEM mode”
Automation brings new ease-of-use capabilities to (S)TEM analysis
In addition to selecting “TEM” mode or “STEM” mode, there are other options to consider when performing (S)TEM analysis. For example, what accelerating voltage do you need to apply to the electron beam? Typical semiconductor applications use electron acceleration voltages ranging between 60kV – 200kV or even 300kV. This can be contrasted with dedicated STEM or STEM-in-SEM configurations which usually do not have such a large range of kV, limiting their resolution and application space. (S)TEM systems offer a wide range of other variables and configurations such as magnification, camera, detector(s) (including a wide variety of 3rd party analytical options). Selecting the optimal parameters and configuration depends on the incoming samples expected and the data you wish to extract from the samples.
Luckily, today’s semiconductor (S)TEM instruments are highly automated, and they make the selection and operation relatively simple allowing a wide variety of imaging and elemental data to be acquired from almost any semiconductor sample type.
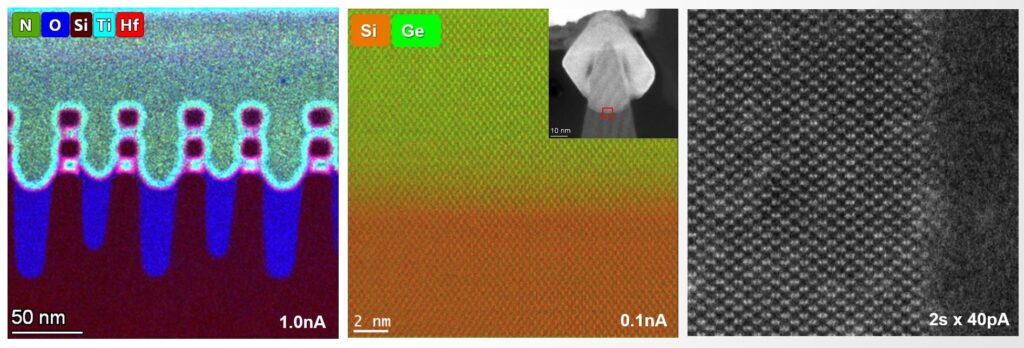
Fig 5: Typical (S)TEM datasets on semiconductor structures show detailed image and elemental data
Another area where automation has had a major impact is in the alignment and calibration of the optical system, which reduces the risk of potential data distortions and artefacts to an absolute minimum.
These factors in conjunction with the system’s ability to calibrate images at the atomic scale, is the reason why (S)TEM is usually relied upon as the source of “reference” data for semiconductor design and fabrication.
The reliance on (S)TEM increases as 3D device complexity increases and critical dimensions shrink – these all drive the need for better characterization and metrology. More EUV lithography steps, atomic interfaces, strain, magnetic fields, and grain orientation are creating the need for ever more reliable analytical data to avoid costly and difficult to control defect mechanisms later in the process yield ramp phase. (S)TEM metrology is now routinely used to provide fast, automated, “ground-truth” reference measurements and characterization for the wafer fab, both to calibrate the fab toolsets, but also to provide rapid and accurate insight into fab process variations. Each of the world’s leading semiconductor manufacturers are now routinely creating tens of thousands of (S)TEM datasets per month (see Fig 6).
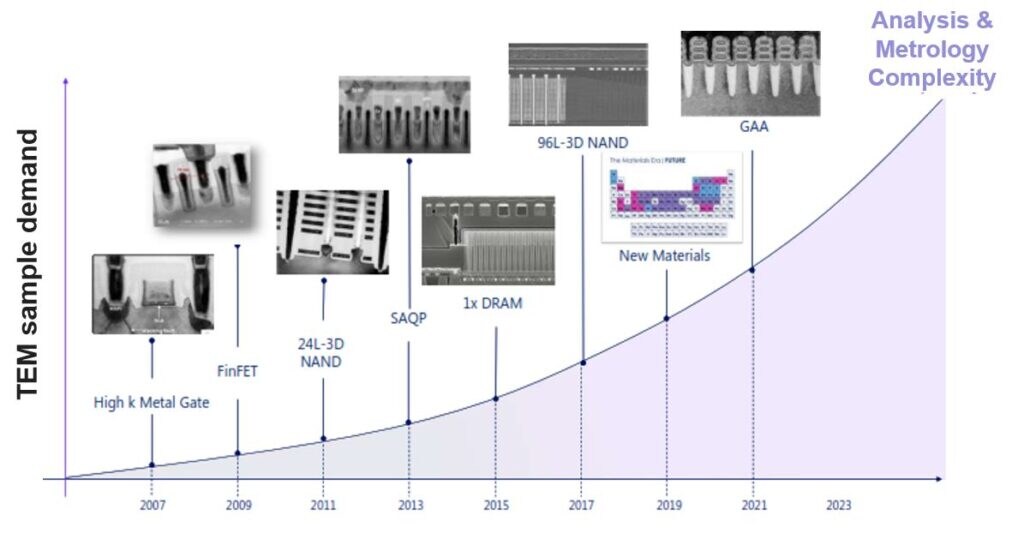
Fig 6: Continued growth in semiconductor TEM analysis and metrology
Understanding the basics of (S)TEM to advance the future of semiconductor technology
In short, (S)TEM analysis has become a near-ubiquitous technique within the semiconductor industry. It provides highly valuable data, accelerating the time to root cause answers and maximizing process yields, particularly in the fabrication of advanced logic and memory devices containing complex, atomically engineered 3D structures such as gate-all-around (GAA) logic transistors, 3D-NAND memory channels and DRAM memory cells.
It’s important that everyone associated with semiconductor analysis and development should understand (S)TEM basics and some of the deep insight that transmission electron microscopy can deliver. To address this need, Thermo Fisher Scientific will present a series of educational content, including this blog series, webinars and more, about the fundamentals of TEM and (S)TEM, including expert insight on elemental analysis, metrology, and other (S)TEM techniques which are now becoming commonplace in the semiconductor industry.
Watch the TEM fundamentals webinar on-demand >>
Our experts look forward to providing you with an understanding of transmission electron microscopy applications and their key role in enabling the next generation of semiconductor innovation.
Also, don’t forget that Thermo Fisher offers a range of live and virtual “hands-on” training courses on all our electron microscopy and defect localization solutions in our worldwide NanoPorts. See here for more details.
//
Paul Kirby is a Senior Marketing Manager at Thermo Fisher Scientific
Leave a Reply